- Bioinformatics
- Bioinstrumentation
- Biomaterials
- Biomechanics
- Biomechatronics
- Biomimetics
- Bionanotechnology
- Biotechnology
- Clinical Engineering
- Bioprocess Engineering
- Medical Imaging
- Neuroengineering
- Ortho. & Rehabilitation
- Pharmaceutical Engineering
- Synthetic Biology
- Systems Biology
- Systems Physiology
- Telehealth
- Tissue Eng. & Regenerative Medicine
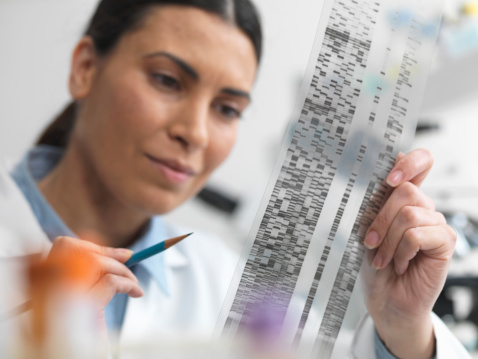
Bioinformatics is focused on finding ways to manage and analyze biological information through the use of computers. The primary goal of this subfield is to increase the understanding of biological processes. Bioengineers working in this area may develop software tools to organize biological data, while utilizing computer science, math, statistics, and engineering.
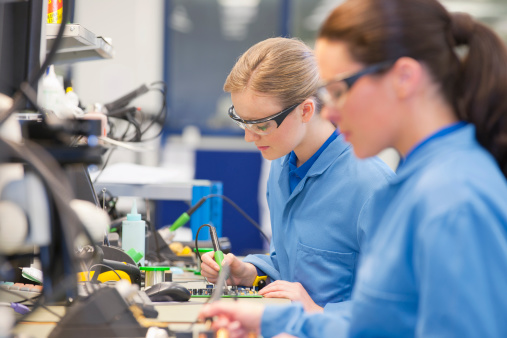
Bioinstrumentation is the application of electronics, computer science and measurements principles and techniques to develop devices used in diagnosis and treatment of disease. Such devices measure physiological levels, including blood pressure and electrical signals from the human body. Bioengineers in this area design, fabricate, test, manufacture and maintain advanced medical instruments and implantable devices including electric sensors, respirators and ultrasound equipment.
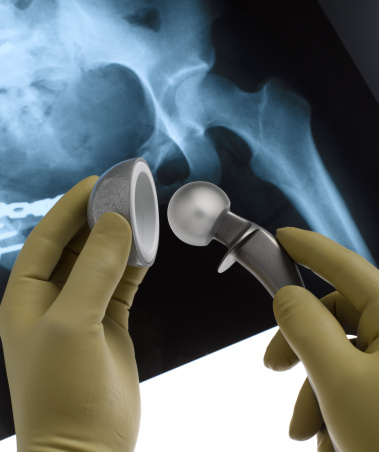
Biomaterials is the study and application of materials (naturally occurring or designed in the lab) that are used in medical devices or in contact with biological systems. Biomaterials can be metals, ceramics, polymers, glasses, carbons, and composite materials. These materials must be fabricated into devices (such as the artificial heart and hip joint) by a biomedical engineer. Engineered materials are increasingly used in medical applications (such as drug delivery, gene therapy, and biomedical and surgical devices) while an understanding of structure-property relationships in natural biomaterials may lead to improved interventions for a wide variety of diseases and injuries.
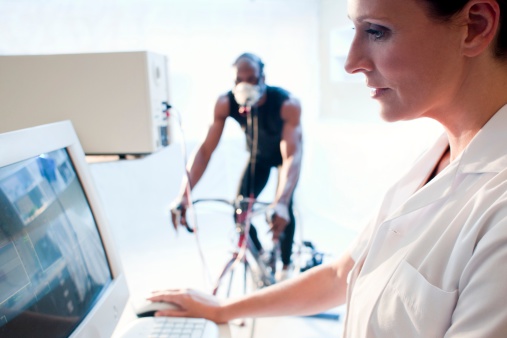
Biomechanics utilizes and applies mechanical principles to explore biological processes and human movement. The study of biomechanics ranges from the inner workings of a cell to the movement and development of limbs, to the mechanical properties of soft tissue and bones. Some biomechanical engineers work to understand how prosthetic devices can be better designed to move with the body, how bodies respond to stress and strain, and how injures can remediated through physical therapy. In sports biomechanics, engineers work in tandem with physicians, physical therapists, athletic trainers, coaches, and athletes to improve performance, improve recovery time, and prevent injury.
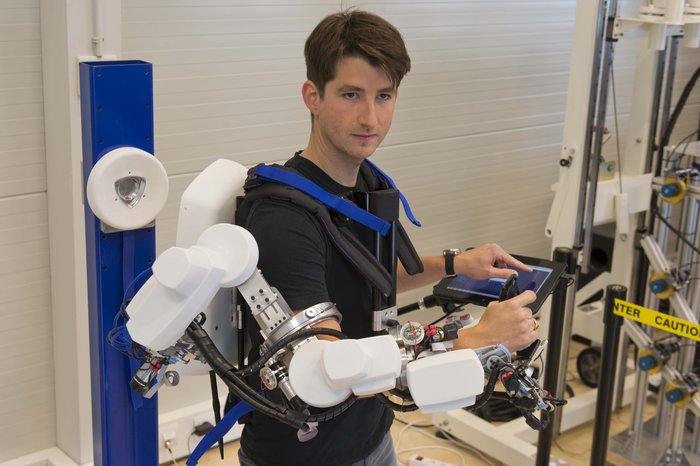
Biomechatronics is one of the newest and rapidly growing subfields of biomedical engineering. Engineers in this subfield design devices that interact with human muscle, skeleton, and nervous systems (such as robotic prosthesis), with the goals of assisting or enhancing human motor control that can be lost or impaired by trauma, disease or birth defects. Biomechantronics is the merging of man and machine in order to augment human performance. The goal of biomechatronic devices is to interface directly with the wearer’s muscle and nervous systems to assist/restore motor control.
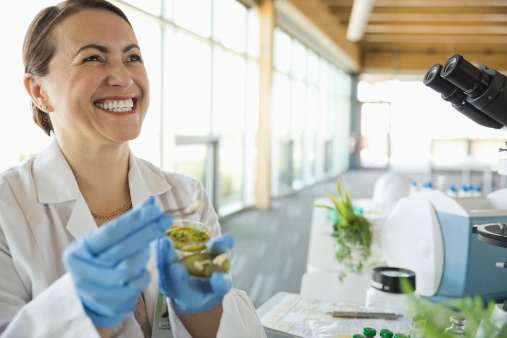
Biomimetics refers to human-made processes, substances, devices or systems that imitate nature. It is the science/engineering of the transfer of ideas from biology to technology, often to solve complex human problems. Brighter screens on medical devices (and cell phones) have come from what researchers have learned from studying iridescent butterflies and beetles, as an example. Biomimetics serves applications in nanotechnology, engineered materials, robotics, artificial intelligence (AI), the medical industry, and the military.
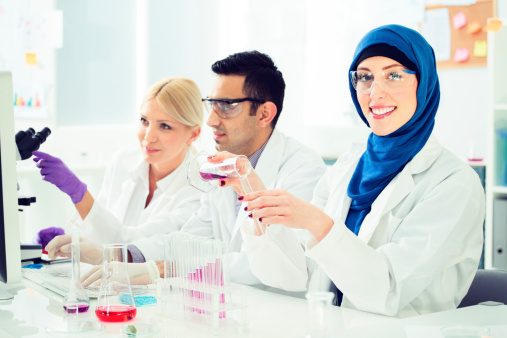
Bionanotechnology takes the nano-scale building blocks of life, such as DNA, to create new tools for science and medicine. It helps us build mechanisms to understand life at the molecular level in order to spur advances in manufacturing, electronics, and medicine.
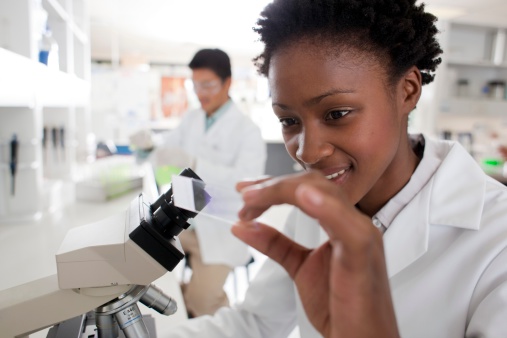
Biotechnology utilizes cellular and biomolecular processes to develop technologies and products that help improve our lives and the health of our planet. Biotechnology products and technologies allow us to have a more abundant and sustainable food supply (agriculture), use safer and more efficient industrial manufacturing (manufacturing), reduce our greenhouse gas footprint (environment), and help us live longer and healthier lives (medicine).
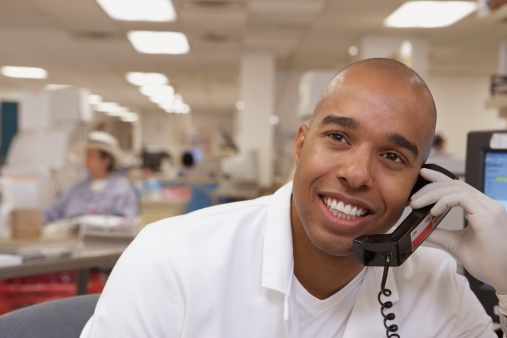
Clinical Engineering is responsible for applying and implementing medical technology to optimize healthcare delivery. Clinical engineers often function as technology managers for medical equipment systems in hospital and health care settings. They work closely with patients, technical and medical staff and administrative staff as well as equipment manufacturers. They also work in industry to assure that new products will meet the needs of tomorrow’s medical practice. They are involved in all aspects of the development process, from medical device design and development, through product sales and support.
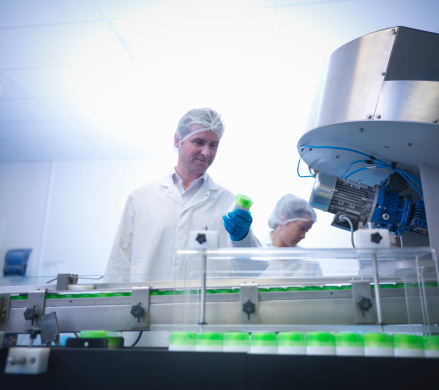
Bioprocess Engineering utilizes biology for cleaner and more efficient bio-manufacturing processes in order to produce food, biofuels, bioproducts, and pharmaceuticals. Bioengineers working in this arena research and develop the biological systems used in the manufacturing of these products, design and test manufacturing equipment and processes, and are involved in the commercialization of products.
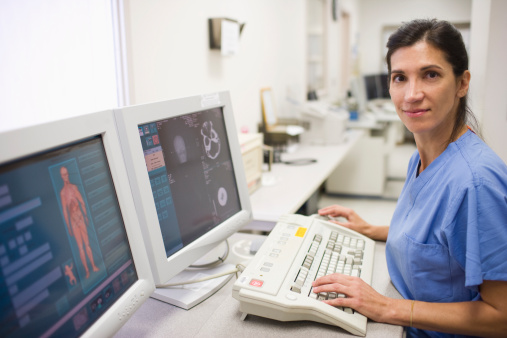
Medical Imaging uses and develops imaging techniques to provide information on two- and three-dimensional structure and function at the molecular, cellular, tissue and organ level in order to understand, diagnose and treat disease. Common medical imaging devices include plain radiography, computed tomography (CT), single-photon emission computed tomography (SPECT), positron emission tomography (PET), magnetic resonance imaging (MRI) and ultrasound imaging (US).
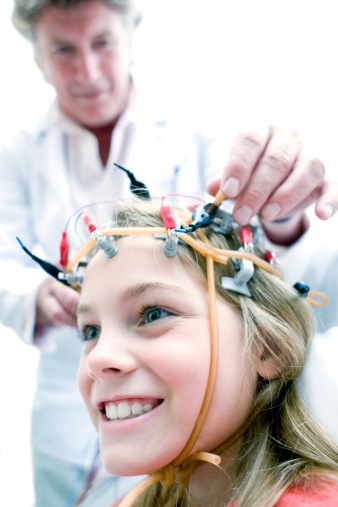
Neuroengineering utilizes engineering tools and strategies to understand, replace, and repair neural systems. A major goal of this subfield is to restore and augment human function by designing medical devices (such as neuroprostheses) that interact with the nervous system.
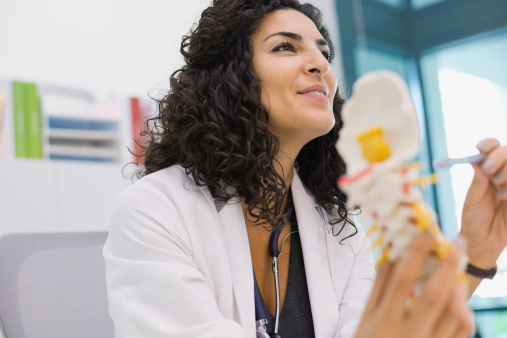
Orthopaedic and Rehabilitation Engineering focuses on studying the effects of injury and disease on the body’s musculoskeletal system in order to improve medical device design. The musculoskeletal system includes the body’s bones, joints, ligaments, tendons, muscles, and nerves and allows you to move, work, and be active. Orthopaedic engineers work to understand cartilage and joint health mechanisms and develop strategies for the prevention and treatment of skeletal joint diseases.
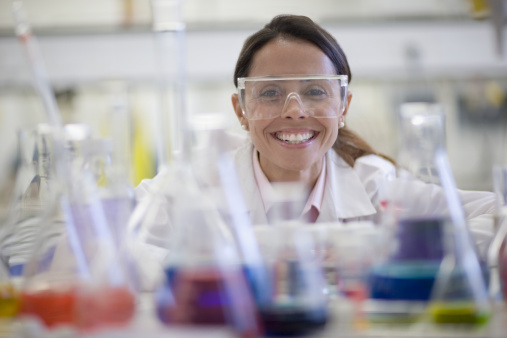
Pharmaceutical Engineering is responsible for the conception and design of pharmaceutical drugs and therapies and for the design and scale up of all aspects of the manufacturing processes needed to commercialize them. Drug discovery is facilitated by pharmaceutical companies, sometimes in partnership with research institutions.
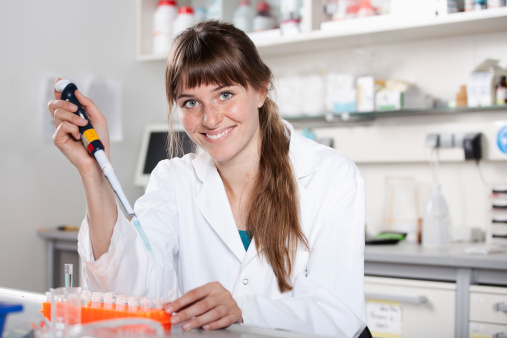
Synthetic Biology incorporates the design and construction of new biological devices, systems, and pathways, as well as the re-design of existing biological systems found in nature. Key technologies utilized in this area include DNA sequencing, gene synthesis, molecular cloning, and modeling. The range of potential applications is vast and includes diagnostics, therapeutics, sensors, environmental remediation, energy production, and a host of other biomolecular and chemical manufacturing outputs.
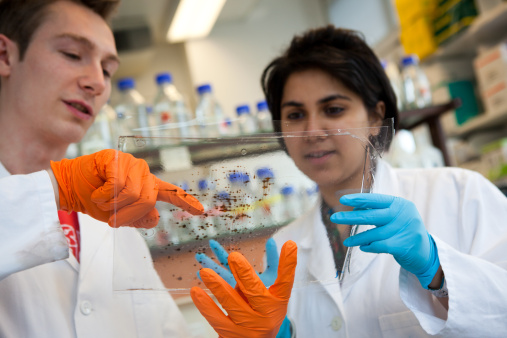
Systems Biology focuses on understanding the structure and function of biological systems on multiple levels—from molecules to organisms—through data garnered from theoretical, experimental, and computational methodologies. It is based on the comprehensive study of the molecular diversity of living systems, both natural and synthetic, the identification of simplifying general principles and patterns that are recurring features in living and engineered systems, and the integration of our biological knowledge in complex models of the regulatory networks that characterize life.
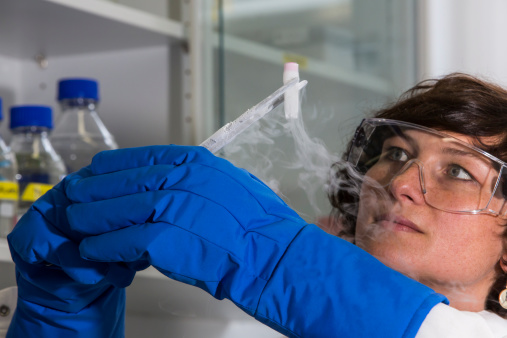
Systems Physiology utilizes engineering tools and strategies to understand how systems within living organisms, from bacteria and humans, function and respond to changes in the external and internal environment. An engineer in this subfield may study how the human body responds to pharmaceutical drugs, as an example.
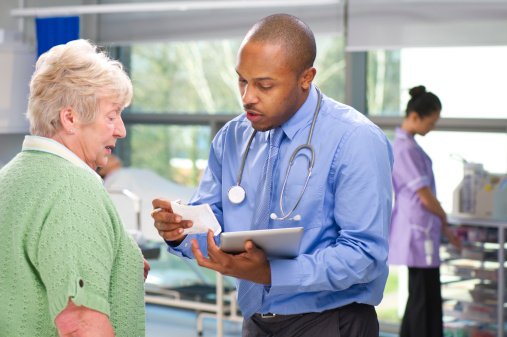
Telehealth utilizes communication technologies to provide and support health care at a distance. A biomedical engineer in this subfield may design medical devices that assess measures of health (such as blood pressure and heart rate) remotely monitored by medical professionals. They may also develop and utilize technologies that transmit patient data while a medical procedure is being performed in order to obtain expert, off-site assistance.
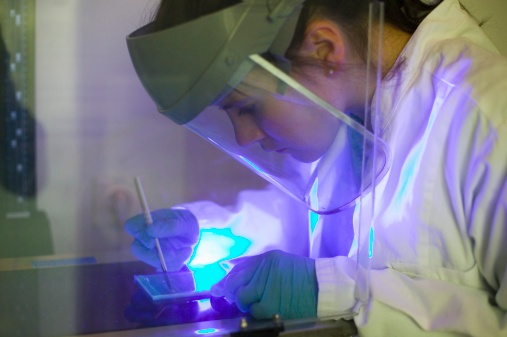
Tissue Engineering and Regenerative Medicine’s objective is to replace, repair, or regenerate tissues and organs in order to treat patients with disease or severe injuries. Bioengineers working in this area create skin substitutes and cartilage and are working to develop a bioartificial pancreas, cardiovascular substitutes such as heart valves and small diameter blood vessels for bypass surgery, treatment strategies for a myocardial infarction, and therapies for a variety of neural injuries and disorders. They apply engineering principles toward the understanding of basic stem cell biology and develop manufacturing processes for the translation of stem cell science.